Passive Design
The basic principle of passive design uses layout, fabric and form to reduce or remove (if possible) mechanical cooling, heating, ventilation and lighting.
The passive design maximises the use of ‘natural’ sources of heating, cooling and ventilation to create comfortable conditions inside buildings. It harnesses environmental conditions such as solar radiation, cool night air and air pressure differences to drive the internal environment. Passive measures do not involve mechanical or electrical systems.
As opposed to ‘active’ design which makes use of active building services systems to create comfortable conditions, such as boilers and chillers, mechanical ventilation, electric lighting, and so on. Buildings will generally include both active and passive measures.
Hybrid systems use active systems to assist passive measures, for example; heat recovery ventilation, solar thermal systems, ground source heat pumps, and so on. Very broadly, where it is possible to do so, designers will aim to maximise the potential of passive measures, before introducing hybrid systems or active systems. This can reduce capital costs and should reduce the energy consumed by the building.
To any passive design, various attributes will be taken into consideration these will cover, but not exclude, Location, Orientation, Massing, Shading, Material selection, Thermal Bridging, Thermal mass, Insulation, Internal layout, Cross Ventilation and Stack Ventilation. This list of design attributes can be endless.
To try and break these large areas into a manageable list, “generally” the flowing areas have been considered to be the “main” 5 principles.
Super Insulated Envelopes
The building envelope is what separates the interior of the building from the exterior; it consists of outside walls, roofs, and floors. In cold climates where inside air is heated to keep the building comfortable, some of that heat will be lost as it moves through the envelope (via the process of conduction). To reduce this heat loss, insulation made of low-conductivity materials is installed within the wall and roof assemblies.
The passive design makes the most of the envelope by super-insulating the building to minimize heat loss. For a Passive design, the aim is to use assemblies with enough insulation to double or triple the heat resistance compared to what is required in current building codes.
The result is a significant increase in the thermal performance expected from the building envelope. Insulating to Passive design levels has the added advantages of greater soundproofing, improved durability, and greater building resiliency—including the ability to maintain interior comfort for extended periods even if there is a power failure.

Achieving Passive design levels of heat resistance is not just about how much insulation you have, but whether that insulation is used effectively. Insulation is most effective when it wraps the building uninterrupted by other materials, but there will always be areas where this is not possible, such as around components used for structural reasons. When a material bypasses the insulation, it is known as a thermal bridge and can significantly reduce the effectiveness of insulation, especially if that material is very conductive, like metal.
The maximum U-value for walls, floors and roofs is 0.15W/m2K, though it is less if the building has an inefficient surface-area-to-floor-area ratio.
Airtight Construction
Heat can also be lost through the envelope via air leakage. A building’s air barrier is a layer of material (membrane, tape, seals) around the envelope that restricts the movement of air in and out of the building. Gaps in the air barrier can allow air to move in and out of the building uncontrolled; they occur when there is insufficient detailing during construction, when there are numerous ducts or other penetrations in the air barrier, or when construction is of generally poor quality.
High volumes of uncontrolled air exchange with the exterior can lead to a whole host of problems, including increased energy use from having to repeatedly reheat the air, discomfort from cold air drafts near the walls, and localized moisture and condensation problems. While air exchange is necessary for ventilation and providing fresh air, it is far more effective to control air exchange by tightening the envelope and using mechanical ventilation.
There are strict design and construction requirements for a Passive design project to be certified airtight. Quantitatively, this means that when tested the building needs to have less than 0.6 air changes per hour to achieve Passive certification.
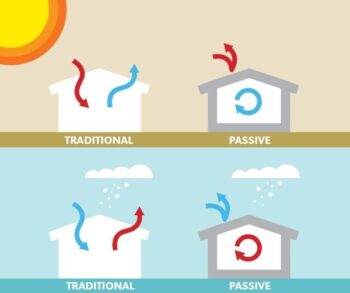
Airtight construction should be tested to a maximum of 0.6 air changes per hour using a blower door at 50 pascals, under both positive and negative pressure. Under current Building Regulations, a dwelling can be completed without testing for air leakage. The regulations allow a leakage rate equivalent to around 15 air changes per hour, which is 25 times worse than Passive design levels. At least one on-site air leakage test must be completed to demonstrate that the building meets the airtightness requirements.
Achieving this degree of airtightness requires careful planning in the design stage, including making sure that the air barrier is continuous and evident on drawings, that effective air barrier materials are used, and that clear detailing for penetrations and terminations is provided. Construction quality with thorough quality control, from the contractor down to the trades, in the installation of the air barrier is critical. The entire construction team should be aware of the important role that airtightness plays in a Passive design project.
Airtight construction on a Passive project will further reduce space-heating costs and localized condensation problems and will provide better comfort inside the building. In a Passive building, these advantages cannot be achieved by tightening the building envelope alone but must be coupled with a suitable ventilation strategy to deal with excess humidity in the building.
An air-tight building will limit heat escape and cut down on the subsequent energy costs, localising any condensation problems and promising the very best comfort within the building.
It should be carefully considered at the design stage, as the air barrier of the building will need to be continuous and clear. It should include all air barrier materials that you plan on using and details of any potential penetrations (and how you will stop them).
Essentially, it means every joint should be perfectly sealed and there should be zero gaps in the ‘skin’ of the build.
High-Performance Glazing
While the walls typically make up the largest area of a building’s façade, the glazing systems (windows and glazed doors) can play an even bigger role when it comes to contributing to space-heating energy. Due to their function (providing light and visibility), glazing systems cannot be insulated to the same degree as a wall, resulting in the windows being the weakest areas of the envelope in terms of heat-flow resistance. Therefore, it is very important that high-performance glazing systems, such as Passive certified windows, are used to reduce that heat flow as much as possible.
Windows and doors usually need to be triple-glazed with a U-value of 0.8W/m2K, averaged between the glass, glazing spacer and frame.
Some key characteristics of a high-performance Passive House glazing system, include nonconductive framing or large thermal breaks; insulated framing; double- or more likely triple-glazed units; argon or krypton gas fill; multiple Low-E coatings; and warm-edge or non-conductive spacers.
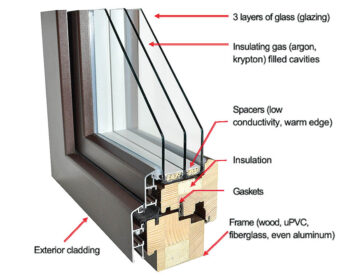
It is important not only to make sure to specify high-performance windows but also to carefully consider how they are incorporated into the building design. Passive designs take advantage of free passive heating from the sun. Solar heat gain through appropriately placed windows can help offset the amount of heat a building needs during colder months.
During the summer months, this needs to be counteracted with shading to prevent too much heat from the sun from getting into the building, causing overheating. For each Passive project, there will be an ideal number of windows that can balance the advantage of free heat from the sun with minimizing the heat loss from having too many windows.
The final consideration for glazing systems is surface temperatures. When outside temperatures are low in winter months, the inside surface temperatures on low-performing windows can also be quite cold. Low temperatures around the window can result in a higher risk of condensation (and potential mould growth) and feeling colder when you are close to the window because of radiant heat loss or from temperature-induced drafts. To reduce these risks, certified windows must be assessed according to hygiene and comfort criteria that set minimum allowable surface temperatures around the window.
Thermal-Bridge-Free
The last envelope consideration is the minimization of thermal bridging. Passive designs also aim to be thermal-bridge-free when it comes to architectural interface details. These are parts of the building where different architectural features meet that require additional attention in construction.
Examples include how a window is attached to the walls, how a wall meets a balcony, and how walls meet at corners. The way these building features connect and are designed can also introduce thermal bridging that’s not always easy to recognize.
Thermal bridging from interface details can have numerous effects on building performance. For highly insulated envelopes like those in Passive projects, thermal bridging can significantly reduce the benefits of super-insulating by allowing heat to flow around the insulation and out of the building, and can also create localized cold spots, increasing the risk of condensation and mould growth around these details.
The easiest way to avoid thermal bridging is by making architectural design changes (where possible), such as using self-supported decks and canopies for low-rise buildings and articulating architecture (lots of corners) on larger buildings.
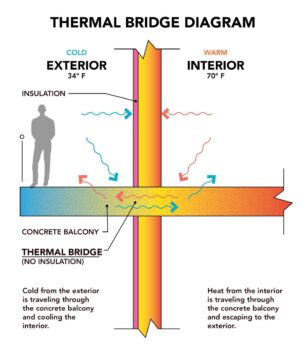
This is not always realistic or achievable, and in these cases, special attention needs to be paid to these interfaces. Reducing direct conductive connections between the interior and exterior is important.
Examples include installing intermittent connections for shelf angles, over insulating in front of certain connections around the foundation, wrapping insulation around protruding details, or using special materials such as thermal breaks.
While it may not seem obvious, the thermal bridges caused by window-to-wall interfaces can have a very large impact. The total perimeter of all the window-to-wall connections can add up to several kilometres on some projects, so how a window is installed into an opening plays an important role in minimizing the heat flow.
Reducing thermal bridging at this connection involves positioning the window to line up with the insulation layer, over insulating in front of the frame, and minimizing how far closure flashings penetrate the rough opening while still maintaining adequate drainage paths. Eliminating or minimizing thermal bridging on Passive projects helps ensure the effectiveness of the envelope performance in reducing space-heating energy use.
All thermal bridges must be less than 0.01W/m2K, known as a psi value; if greater, they must be included in the calculation. Thermal bridges are weaknesses in the insulation where it is not continuous, such as wall ties and junctions. They often occur at internal corners, resulting in condensation and mould growth. The Building Regulations allow bridges 15 times worse than Passive levels.
Heat Recovery Ventilation
Since Passive projects are airtight, a ventilation system is needed to bring in the fresh air and exhaust out built-up pollutants, odours, CO2, and moisture. During winter, this means dumping out warm air and bringing in cooler air that needs to be heated up again, which increases the energy. A Passive ventilation system uses a heat recovery ventilator (HRV) to continuously remove stale or moist air and deliver fresh air. During this process, it extracts heat from the exhaust air and puts it into the incoming air without directly mixing the airstreams. This way, all the heat in the exhaust air is not completely lost to the outside. For a Passive House HRV, at least 75% of that heat needs to be recovered.
For warmer summer months, most Passive certified ventilation systems also feature a summer bypass damper that diverts air around the heat recovery core. That way the system can still bring in fresh air but doesn’t recover heat when it’s not needed.
In dry locations, like the prairies, buildings without humidification in winter can leave the interior spaces at low interior humidity (under 30% RH), which leads to discomfort, potential health issues, and damage to interior materials.
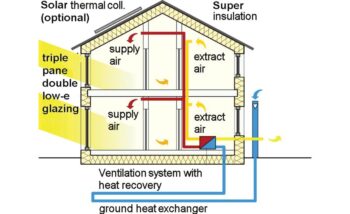
In these cases, an energy recovery ventilator (ERV) can be used. Unlike HRVs, which only transfer heat, ERVs can also transfer moisture from the outgoing exhaust to help maintain more-comfortable moisture levels in interior spaces. Occupants can also utilize natural ventilation (using cool summer breezes) from opening windows to exchange stale air by nonmechanical means and are encouraged to do so when it makes sense.
Passive designs utilize both methods to keep ventilation energy to a minimum. While Passive projects can still be fitted with a heating system (such as air source heat pumps, electric baseboards, or boilers) having heat recovery in ventilation can greatly reduce the size, capacity, and maintenance needs of this equipment, shifting project costs from the mechanical systems to a superior building envelope.
Overheating is prevented, with temperatures above 25°C occurring for no more than 10% of the year. Heating may be provided simply by warming the ventilated air or using any efficient system. Following the Passive principles will result in a building with a maximum heating demand of 15kWh/m2 annually, or a heating load of 10W/m2. To put this into perspective, a typical three-bedroom UK house uses around 180kWh/m2 a year and still fails to provide comfort and sufficient fresh air.
Visited 390 Times, 4 Visits today